While some of the symptoms and outward signs of autism spectrum disorders (ASD)—such as deficits in social interactions, inappropriate responses to sensory stimulation, and delays in acquiring language skills—are commonly recognized, even stereotyped, the changes in the brain that underlie these complex behavioral abnormalities haven’t been well understood.
But findings in a recent study, completed by Katie Fricano-Kugler, PhD, and Bryan Luikart, PhD, with a team of researchers at Dartmouth’s Geisel School of Medicine and published in the journal Biological Psychiatry, are providing further insight into the neurobiological basis of these disorders.
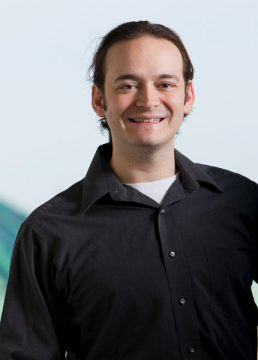
“We’ve been working to understand how mutations in the gene PTEN, which have been found in patients with ASD, affect neuronal development,” explains Luikart, an associate professor of molecular and systems biology at Geisel and lead author on the study. The Luikart Lab is focused on understanding the molecular mechanisms that direct the formation of synapses onto new neurons as they integrate into the synaptic circuitry of the central nervous system.
The PTEN gene normally functions to control cell growth and also regulates the ability of neurons to alter the strength of their connections. “In previous studies, we found that removal of this gene in mice results in the overgrowth of neurons leading to hyper-connected neural circuits,” Luikart says. “We therefore believe that this neuronal circuit hyper-connectivity could be the fundamental basis for the symptoms exhibited by ASD patients.”
In their new study, Luikart and his team sought to determine if the mutations found in patients would cause the same type of neuronal overgrowth that is seen when the normal healthy gene is completely removed from the neurons of mice.
To do this, they established a method to mimic the genetic defects found in human autism patients—by engineering viruses to “knock out” the normal mouse PTEN gene and replace it with the mutated human PTEN gene—and then used sophisticated imaging and electrophysiological techniques to study how neuronal function was altered in the mice.
“We found that putting human PTEN-bearing mutations that have been identified in patients into the neurons of living mice indeed results in the neurons growing abnormally large,” he says, “proving that ASD-associated mutations in the PTEN gene make it unable to regulate the normal growth of neurons in the living mouse brain.”
In addition, the researchers identified a type of mutation in PTEN that causes the protein to no longer function in the nucleus of the cell.
“Previously, it was believed that PTEN at the cell membrane regulates cellular growth,” says Luikart. “While this remains true, we determined that PTEN in the nucleus is also capable of regulating cellular growth. Importantly, we found that if we could force the ASD-associated mutant PTEN into the nucleus of the cell, we could partially restore the size of the neuron.
“This discovery gives us new insight into how PTEN works to regulate neuronal growth, and suggests that a strategy regulating the location of PTEN within cells could be therapeutic for autism spectrum disorders.”